Segmented 3D Trap
The segmented ion trap is used to perform quantum state control and quantum information processing using both beryllium and calcium qubits, with a particular focus on scalable methods for quantum computing. We use an ion trap with 15 segments, which allows shuttling of ions along the trap axis. Trapped ion internal and motional states are controlled using laser radiation. For beryllium ion qubits, the primary control is a Raman laser system at 313 nm. For calcium ions, the primary control laser is a narrow-linewidth optical source at 729 nm.
A key element of this setup is that it has been used as a test bench and development environment for a state-of-the-art experimental control system featuring advanced in-sequence feedback capabilities, which we take advantage of across all experiments in the group.
Research performed in this setup splits into three directions:
Quantum harmonic oscillator control
The trapped charged ion oscillates about its equilibrium position, forming a near-ideal quantum harmonic oscillator. We use lasers to control this motion in order to create, control and analyse quantum states. Recent results include the use of reservoir engineering to create squeezed states, the generation of some of the largest Schrödinger’s cat states which have been generated in the laboratory, and the realization of a logical qubit encoded in a harmonic oscillator. Since the states we have generated are among the largest states of the harmonic oscillator realized to date, diagnosing these states has required new measurement techniques. The Wigner function of a Schrodinger’s cat state is shown in the picture.
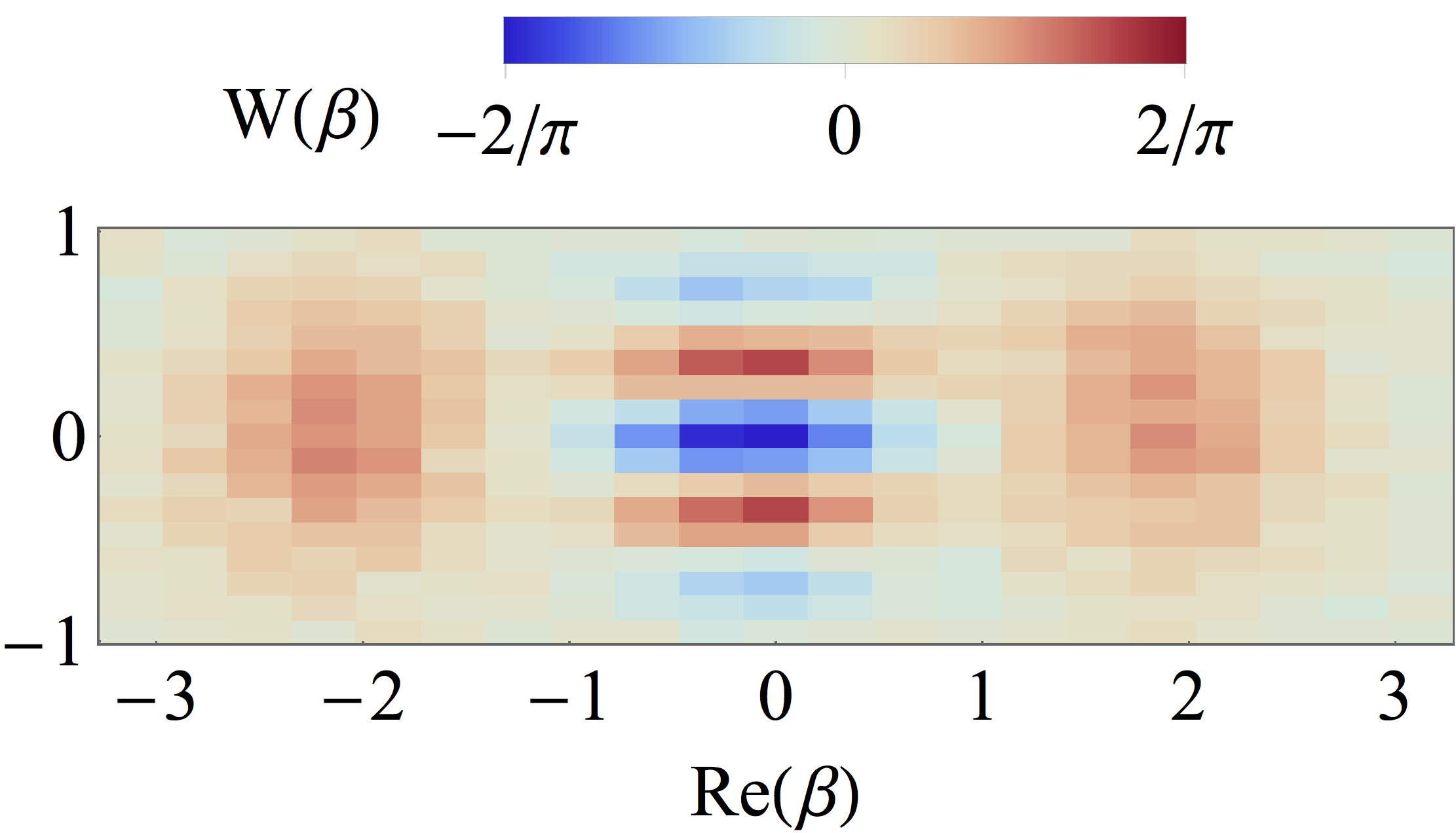
Mixed species quantum computing
Operation of ion traps with multiple ion species is thought to be key to realizing large-scale ion-trap quantum computation. The primary advantage that this offers is that resonant light can be scattered from one species while quantum information stored in the other is preserved. This allows the ion motion of “qubit” ions to be re-cooled by laser cooling on the co-trapped “coolant”, but also allows detection of one species with no crosstalk on the other. The latter is particularly useful in quantum error correction, which requires repetitive readout of ancilla qubits while maintaining information stored in the quantum register. We were recently able to demonstrate such repeated readout and correction on a two-ion beryllium qubit register using a calcium ancilla.
Ongoing work relates to implementing more complex quantum protocols, optimising fidelities, and improving and understanding limits to our implementation of splitting and recombining dual-species ion chains, along the lines of the quantum-CCD architecture.
Transport quantum logic gates
One of the key issues for scaling quantum computers is the scalable implementation of laser-driven gates. In this work we attempt to realize gates by moving ions through static laser beams rather than by pulsing lasers on and off, where we were able to show parallel operations in two parts of the trap simultaneously. This led us to develop methods for characterizing general time-dependent Hamiltonians, which also allowed us to characterize and optimize ion transport.
This work is supported by external grants from the SNF, ETHZ, and IARPA.